JOTS v26n2 - The Strategic Thinking of Novice Designers: Discontinuity Between Theory and Practice
The Strategic Thinking of Novice Designers: Discontinuity Between Theory and Practice
Malcolm Welch and Hee Sook Lim
Introduction
In two studies we conducted, untutored Grade 7 students produced solutions to a design-and-make task in ways significantly different than ones prescribed by many textbooks and theories about learning to design. We found that novice designers (a) sequence the subprocesses of designing quite differently than the prescribed models, (b) do not generate several possible solutions and choose the most effective, (c) make greater use of three-dimensional modeling, (d) use less two-dimensional modeling than suggested by textbooks, and (e) constantly evaluate their design proposal from the earliest moments of the design-and-make process.
The first study addressed the question: What design processes do Grade 7 students who have received no prior instruction use to produce a solution to a design-and-make task? Since the strategies used by these students may have been a function of the particular task and the way it was presented, a follow-up study addressed the question: Is the design process used by novices dependent on the task? This second study provided an opportunity to further investigate protocol analysis as a method for understanding novice designers' strategies. It also resulted in the refinement of a coding scheme to describe design process skills.
This article first describes the theoretical framework used for the two studies and reviews related literature. Next, the methods used to collect and analyze the data are described. This is followed by a discussion of the strategies used by students and how the strategies differ from those in theoretical models of the design process. The implications of these findings for the teaching of design and technology complete the article.
The Centrality of Designing
Much current school work presents tasks to students in a form that assumes there is only one correct way to do it and often only one correct solution. Design and technology education, however, presents tasks that have many possible solutions. Furthermore, it provides students with opportunities to apply knowledge to generate and construct meaning. It fosters the kind of cognition that combines declarative knowledge, the what , with procedural knowledge, the how . As Kimbell, Stables, Wheeler, Wosniak, and Kelly ( 1991 ) pointed out, "there [is] general agreement on certain basic tenets of [technology education]. It is an active study, involving the purposeful pursuit of a task to some form of resolution that results in improvement (for someone) in the made world" ( p. 17 ). And as Breckon ( 1995 ) reiterated, "technology [education] provides that excellent method of learning-learning through doing" ( p. 11 ).
The "doing" in technology education involves using design process skills to design and make an artifact in response to a need. A typical form of design process includes identifying needs and opportunities, understanding and detailing a problem, generating possible solutions, building a solution, and evaluating a solution. This process shares many properties with a general problem-solving model used in the resolution of ill-structured problems ( Simon, 1973 ).
According to Jones ( 1970 ), "all [models of the design process] are attempts to make public the hitherto private thinking of designers, to externalize the design process" ( p. 3 ). This is nearly always accomplished by using a diagram to show the steps in the process and the relationships between them. Siraj-Blatchford ( 1993 ) noted that "providing a simplified model of the process of design which teachers may adopt heuristically provides for the student what Bruner ( 1986 ) has termed scaffolding" ( p. 22 ). Vygotsky ( 1986 ) referred to this period-when the teacher does for the student what they are not yet able to do for themselves-as the "zone of proximal development" ( p. 33 ), the gap between what an individual can do alone and unaided, and what can be achieved with the help of more knowledgeable others ( Bennett, 1992 ). For as Schn ( 1987 ) pointed out, one of the difficulties for the novice designer is that
designing is a holistic skill [which] one must grasp as a whole in order to grasp it at all. Therefore one cannot learn it in a molecular way, by learning first to carry out smaller units of activity and then to string those units together in a whole design process; for the pieces tend to interact with one another and to derive their meanings from the whole process in which they are embedded [Nevertheless], it is true that design processes may be broken into component parts by strategies of decomposition useful both to practice and to coaching. ( pp. 158-159 )
Models of the design process are readily available in both the technology education literature and school textbooks, and a number of authors have provided detailed historical accounts of their development (e.g., Johnsey, 1995a ; Welch, 1996 ). A recent model "reject[s] the idea of describing the [design] activity in terms of the products that result from it, and instead concentrate[s] on the thinking and decision-making processes that result in these products" ( Kimbell et al., 1991, p. 20 ). The essence of this model is that ideas conceived in the mind need to be expressed in concrete form before they can be examined to see how useful they are. In other words, "the interrelationship between modelling ideas in the mind and modelling ideas in reality is the cornerstone of capability in technology" ( Kimbell et al., 1991 p. 21 ). Yet as Johnsey ( 1995a ) suggested, "the model is [purposely] vague about what might be happening at any point in the process" ( p. 207 ), reminding us of Lawson's ( 1990 ) observation that, in attempting to describe how designers design, "there is not a great deal of action to be seen it is what goes on in the designer's mind which really matters" ( p. 24 ). Perhaps it is because so much of the designer's work is hidden that few studies have attempted to investigate their actual practice. Studies of expert designers ( Akin, 1978 ; Darke, 1979 ; Eastman, 1970 ; Schn, 1983 ) have provided empirical descriptions and models. Recent studies of novice designers at the elementary level ( Johnsey, 1995b ; Outterside, 1993 ; Roden, 1995 ), at the secondary level ( Kimbell et al., 1991 ), and at the university level ( Elmer, 1996 ) are beginning to provide useful insights. Yet an enhanced understanding of the strategies of untutored students would undoubtedly exert some good influence on teaching. Hence the next section of this paper describes a method developed to investigate the strategies used by untutored designers.
Method
Ill-structured problem solving has been investigated using protocol analysis (e.g., Ericsson & Simon, 1984 ). According to Hayes and Flower ( 1980 ), a protocol is "a description of the activities, ordered in time, which a subject engages on while performing a task" ( p. 4 ). In the two studies reported in this paper, verbatim transcripts (from audiotape recordings) of the naturally occurring conversation between students as they were designing and making provided the protocols. Viewing the activity of "designing and making" as a particular form of problem solving allows for the adoption of protocol analysis as a research method in this study. Data were provided through the direct observation of novice designers as well as retrospective interviews with them.
The first of the two studies described here involved ten Grade 7 students working in single-sex dyads. Previous research with dyads ( Meyer, 1991 ) found that while those of mixed gender often do not communicate well or work cooperatively, "the use of single-sex dyads encourage[s] students' conversation as a means to make their thinking explicit" ( Meyer, 1991, p. 14 ). Students were required to design and make a solution to a task entitled "Paper Tower." The design brief read as follows:
Using ONE sheet of 220 mm x 280 mm white paper and 100 mm of clear tape, construct the tallest possible tower. You will also be given pink paper. This you may use in any way as you develop your solution. However, NONE of the pink paper may be used in the tower you submit as a final product.
Limitations: There is a time limit of one hour. The tower must be free standing. It cannot be taped to the floor nor to anything else. When you have finished, the tower must stand for 30 seconds before having its height measured.
It is possible that the strategies used by students to generate a design proposal may have been a function of this particular design brief, the way it was presented, and the tools and materials available to produce a solution. To rectify that possible bias, we conducted a follow-up study of eight Grade 7 students, again working in single-sex dyads. They were given two hours to complete a different task using a wider range of tools and materials. Each dyad was given the following design brief:
The Context: Your parents have invited your uncle, aunt, and five-year-old cousin to visit and stay with you for two weeks. It so happens that your cousin's birthday falls on the second day of the visit. You want to give him/her a birthday present but, unfortunately, you are too short of money to buy one. So you have decided to make something as a surprise. You know that your cousin enjoys playing with toys that move, so you have decided to design and make one. Not only does this solve the problem created by having no money, but it offers the opportunity to give your cousin something really special-a toy you have designed and made. Design brief: Design and make a moving toy that will amuse and intrigue a five-year-old boy or girl.
In both studies the students' designing and making was videotaped and audiotaped. The natural talk between the subjects was transcribed verbatim. A semi structured retrospective interview, conducted with each dyad as they watched the videotape of themselves during the designing and making session, was also transcribed. (For a detailed description of the method used, see Welch, 1996 , 1998 ).
Transcripts of the natural talk during the design-and-make session were segmented into speech bursts. A speech burst was defined as "a complete portion of text uttered by a subject without interruption from that subject's partner" ( Welch, 1996, p. 43 ). A description of the subjects' actions was added to the right of each segment ( Figure 1 shows how students' speeches were translated into design strategies.)
The time at which a change in the subjects' actions occurred was added to the left of each segment, thus allowing calculation of the duration of each period of action. A coding scheme (see Table 1 ) was used to code actions of the subjects. Those actions coded as designing and making were analyzed using descriptive statistics. This analysis provided the data for "mapping," using an XY scattergraph, the design strategy of each dyad. These maps provided a visual representation of the design process used by each dyad, which permitted a comparison between dyads in this study, between dyads in the two studies, and between all nine dyads and a map of a theoretical model derived from the literature (see Welch, 1996 ).
Results
Figure 2 represents the strategies used by the five dyads in the first study. The map shows quite clearly the dominance of three-dimensional modeling throughout the entire period when students were developing a solution. Equally clear is the iterative relationship between evaluating and modeling.
The map also shows how little time was spent at the beginning developing a solution by discussion or drawing and how quickly students moved to modeling with three-dimensional materials.
Figure 3 shows the sequence in which Dyad 1 in the follow-up study employed elements of the theoretical model of the design process. It offers a typical example of all four dyads in the second study. The similarities between Figure 2 and Figure 3 are striking, including (a) the large proportion of time devoted to three-dimensional modeling; (b) the small amount of time spent generating alternative solutions, either by drawing or discussion; (c) the almost immediate move to three-dimensional modeling to develop ideas; and (d) the frequency and consistency with which the developing solution is evaluated.
15,58 S16: "Like something that's like strategy
and needs thinking so it doesn't get
bored. Something."391
392
393Discussing possible
solution.16,04 S15: "Um, so a board game or " 395 16,05 S16: "Yeah, we could make a board game,
but its on a tray, right?"397
398Refers to performance
criteria contained
in design brief.S15: "Yeah." 400 16,40 S16: "And he needs to do it by himself." 402 14,41 S15; "We could use this stuff." 404 Picks up card.
Subjects examine
materials.S16: "Like what?" 406 16,55 S15: "For the board, but also we could use
this, he has on a tray."408
40916,56 S16: "He has to play by himself though,
right?"411
412Refers to
performance criteria.17,03 S15: " , oh yeah, so I guess let's just make
a toy."414
415Looks at materials
on table.Figure 1. Sample of a segmented protocol. When the strategy used by each of the nine dyads is compared to a map of the theoretical design process ( Figure 4 ), five significant differences are evident.
First, students' strategies are more complex than suggested by any of the models. They did not work in a linear way through the steps identified in textbook models. Understanding the problem appeared to emerge from an exploration of solutions. Students moved very quickly to solution generation. Students did not appreciate the importance of analyzing and focusing on the problem before "jumping straight to design ideas" ( Harding, 1995, p. 19 ). Modeling was shown to be a complex activity, more accurately described by a model-test-refine-test iteration. This iteration itself appears to act as a source of inspiration for new sketching by Dyad 1. The model was the prototype for solutions. Evaluation occurred not as a summative activity after generating and modeling and building, but as an integral and ongoing activity.
Second, these students did not sketch several possible solutions to evaluate their merits. Sketching played an especially small part in the development of a solution. Nor was sketching viewed as a necessary first step in the development of a solution.
Third, it appears that the preferred strategy for developing ideas is modeling in three-dimensional form ( Welch, 1998 ). Students moved to modeling much sooner than predicted by textbook models. The evidence suggests that novice designers are anxious to begin modeling, even before a solution has been fully worked out. This modeling served several purposes: externalizing ideas; providing a method of testing, refining, and evaluating ideas; and stimulating new ideas. Modeling appeared to be an essential stimulus to the ongoing development of ideas.
Fourth, constant evaluation was an integral and ongoing activity while students were designing. Evaluating occurred consistently from the earliest moments of designing.
Fifth, and finally, students in the second study made no distintion between modeling a solution and building a prototype. Except for a brief period of sketching by Dyad 1, the model was the prototype for these students.
Discussion
The results reveal good reason to doubt the efficacy of requiring students to follow any form of a linear or sequential design process model, as found in many textbooks and curricular documents. Our studies revealed that untutored designers do engage in many of the subprocesses of theoretical models but do not prioritize or sequence these subprocesses as suggested by the models. This suggests a need for teachers to explicitly teach process skills that will assist students' designing but which do not impose a strict sequence in which those skills are applied. Recent research by Stables ( 1997 ) also noted "the importance of children working in a responsive, rather than a prescriptive, manner when engaged in designing and making" ( p. 11 ). Yet at the same time, as Kimbell ( 1990 ) described, students must be provided with a superstructure to begin designing. They must be able to think and work strategically, so when time runs out at the end of a project, they are where they want to be.
Metacognitive skills may be central to students' technological capability. Metacognition is knowledge about thought processes and how to monitor, control, and evaluate one's performance on cognitively demanding tasks ( Sternberg, 1994 ). Most approaches to teaching of thinking and problem solving now put some emphasis on metacognition ( Presseisen, 1987 ). As De Miranda ( 1998 ) noted, "technology education requires that the learner be highly active in the learning process and exercise considerable control in monitoring [his or her] own progress in accord with metacognitive processes" ( p. 15 ). And Resnick ( 1987 ) claimed that if higher order thinking skills are to be an outcome of teaching, instruction must be metacognitively aware and informed. Metacognitively aware instruction, according to De Miranda ( 1998 ), "attempts to transfer cognitive functions from the teacher to the student" ( p. 2 ).
Table 1 Codes to Describe Designing and Making
Step Code Definition
Understanding the
problem
Generating
possible solutions
Modeling a
possible solution
Building a
prototype
EvaluationRBRF
DERF
DCONS
GEN
DRAW
PMU
MANIP
MMU
RMU
CMMU
ARM
ABAN
PPR
MPR
IPPR
MODPR
EGEN
EDRAW
TMU
EMU
TPR
EPR
RRMU
RRPR
Reading design brief as given to subjects by researcher
Discussing/referring to performance criteria
Discussing/referring to constraints
Discussing possible solution
Sketching/drawing possible solution
Planning the making of a mock-up
Manipulating materials to explore one element of a possible solution
Making a mock-up
Refining a mock-up: making modifications to current solution
Making a copy of a previous mock-up
Checking available resources and materials
Abandon current solution: begin new solution
Planning the production of a prototype
Making a prototype
Identifying a problem with a prototype
Modifying and improving the prototype in terms of the original need:
i.e., making a design change
Evaluating as subjects talk about a possible solution
Evaluating as subjects talk about a sketch or drawing
Testing one element of a mock-up in terms of the design brief
Evaluating mock-up on terms of design brief
Testing one element of the prototype as making continues
Evaluating the prototype in terms of the design brief
Recording results from mock-up
Recording results from prototype
![]()
Figure 2. The Strategy used by Dyad 5 in Study 1.
![]()
Figure 3. The Strategy used by Dyad 1 in Study 2. Schoenfeld ( 1987 ), in a summary of the research on metacognition, identified three related but distinct categories of intellectual behavior associated with metacognition, each of which has importance for teaching and learning in design and technology education:
- Knowledge about one's own thought processes. How accurate is the student in describing his or her own thinking?
- Control, or self-regulation. How well do students keep track of what they are doing when designing and making, and how well do students use the input from these observations to guide their actions?
- Beliefs and intuitions. What ideas about technology (designing and making) does the student bring to the task, and how does this shape the way he or she goes about designing and making?
The first two categories, knowledge about one's own thought processes and control, or self-regulation, are germane to the results of the two research studies reported here.
According to Schoenfeld ( 1987 ), students are not very good at describing their own mental abilities. Yet teaching students this metacognitive skill is important. First, good study skills depend, in part, on students' abilities to make realistic assessments of what they can learn because successful designing and making require students to effectively use what they know.
The second aspect of metacognition, control or self-regulation, may be thought of as a management issue. How well are students able to manage their time and effort as they engage in designing and making? This management has several components, including (a) making sure that one understands what a problem is all about before hastily attempting a solution, (b) planning, (c) and monitoring progress while allocating resources wisely as one works on the problem.
In his research with mathematics students, Schoenfeld ( 1987 ) showed how the absence of the skill of self-regulation can have "disastrous consequences" ( p. 193 ) when students are problem solving. In Schoenfeld's research, students read a mathematics problem, made a correct conjecture, then made some mistakes and became "bogged down in the calculations" ( p. 193 ). In Schoenfeld's words, "the students spent twenty minutes on a wild goose chase" ( p. 193 ).
![]()
Figure 4. Map of the five-step theoretical design process used in this study ( Welch, 1996 ). In a similar way, Dyad 2 in the first study read the design brief (albeit too quickly to fully understand the task instructions), decided upon a poor solution (using the wrong materials), and then persevered with it to the exclusion of all other possibilities. The result was a failure: a short tower that would not stand for 30 seconds. This failure to successfully create a solution may have been in part because the students lacked the metacognitive skills of self-regulation and monitoring. Teaching these skills would have enabled the students to better access and manage their task-relevant knowledge.
But the failure on the part of Dyad 2 to create a satisfactory solution may also reflect a difference between novice and expert problem solvers. The failure of the solution was in part a function of the rapidity with which the students moved to solution generation. This finding is supported by research on expert/novice problem solving, which has shown that at the beginning of a problem-solving episode, experts spend more time attempting to "understand" the problem, whereas novices move more quickly to solution generation ( Chi, Glaser, & Farr, 1988 ). Novice problem solvers spend far more time doing rather than thinking or planning, neither analyzing the task adequately nor monitoring their own performance. The inability (or reluctance) to consider more than one solution to a design problem is highly problematic. As Bruner ( 1966 ) pointed out, "learning and problem solving depend upon the exploration of alternatives" ( p. 43 ).
A second significant result was the critical role modeling (in three-dimensional materials) played in students' thinking. Modeling was used to support a range of activities: increasing understanding of the problem, stimulating the generation of solutions, seeing what a design would look like, testing, and continuously incorporating modifications and improvements into a solution ( Welch, 1998 ). Yet this result contradicts the strategy proposed by most design process models: that students sketch several possible solutions before moving to modeling in three-dimensional materials. Clearly, the results of these two studies suggest that teachers must think carefully about the teaching of two-dimensional and three-dimensional modeling skills. It appears important to provide students, early in the process, an opportunity to explore, develop, and communicate aspects of their design proposals by modeling their ideas in three-dimensional form. Furthermore, a teacher's continued insistence that students generate several design ideas, the "three-ideas paradigm" ( Kimbell, 1997, p. 2 ), may be counter-productive ( Hennessy, McCormick, & Murphy, 1993 ).
In both studies, modeling was seen to be a complex activity, more accurately described by a model-test-refine-test iteration. The following example, taken from the transcript of Dyad 4 in Study 1, illustrates the point.
Students S7 and S8 had previously rolled and taped one sheet of paper into a single cylinder 280 millimetres tall. S8 began to discuss (GEN) how a single sheet of paper could be cut into two strips, each of which could be rolled into a cylinder before combining the two cylinders:
S8: "You could cut it and then roll 174 half of it and roll the other half and 175 stick it together to make it tall." 176 Her partner agreed: S7: "Oh yeah, try it." 178 S8 cut the paper into two equal pieces, each 140 x
220 millimetres (Model). Each student then rolled and
taped one piece into a cylinder (Model).S7: "How's this?" 182 S8: "Roll it this way." 184 S7: "Tape the side so it will stay." 186 "Here." 187 "We'll tape the bottom together." 189 S8 then took the cylinder made by S7 and joined the
two together (Model).S8: "Okay, yours is strong so we can 191 stick it, I'll just " 192 S7: "I hope it stands. This won't, no, 194 this won't stand up." 195 (Attempts to stand one section - Test) S8: " put a little tape." 197 S7: "Okay, will it stand?" 199 S7 attempted unsuccessfully to stand the tower (Evalu-
ate). S8 identified what she thought was the problem:S8: "I just got to make it even on the 201 bottom." 202 S8 used scissors to trim the bottom edge of the
tower (Refine). S7 made a second unsuccessful
attempt to stand the tower (Evaluate). S8 again
used the scissors to trim the bottom edge
(Refine). The next attempt to stand the tower
was successful (Evaluate) and so S7 measured
its height (Evaluate).This example provides clear evidence of a model-test-refine-test iteration. Figure 5 shows the sequence graphically.
The data also show that subjects frequently repeated the test-refine-test part of the loop before returning to modeling. This sequence of activities may be an important aspect of the behavior of untutored designers because modeling appeared to increase students' understanding of the problem, catalyze additional solutions, help refine their ideas, increase exploration of the properties of materials further, and increase students' practice of tool skills.
This model-test-refine-test strategy parallels that of the bricoleur ( Levi-Strauss, 1968 ), the designer who constructs a solution by arranging and rearranging, by negotiating and renegotiating with a set of well-known materials The bricoleur resembles the painter who stands back between brush strokes, looks at the canvas, and only after this contemplation, decides what to do next. ( Turkle & Papert, 1990, p. 352 )
The subjects in these two studies operated as bricoleurs , beginning with a simple solution and shaping it gradually by successive modifications. If a change did not work, it was undone and replaced with another small change. Schn ( 1987 ) captured the richness of this experience when he wrote "designing is a creative activity. A designer's reflective conversation with the materials of a situation can yield new discoveries, meanings, and inventions" ( p. 161 ).
The absence of a distinction between modeling and prototyping by students in the second study indicates the importance of the form in which tasks are presented to students. The task in Study 1 clearly indicated the need to differentiate between a "developing solution" and a "final product." Additionally, different materials were provided for the solution and the product. In Study 2 no such distinction was made. Making was an ongoing part of the process, fully integrated with other design process skills.
The two studies also identified the crucial role evaluation plays as students design. Thus teachers need to stress the importance of ongoing evaluation since it is likely to increase the quality of both the end product and the ability of the student to design effectively. The recognition of a model-test-refine-test iteration so dominant in the strategies used by subjects should, as Johnsey ( 1995b ) has also found, encourage teachers to take a broader view of the nature and role of evaluating when students are designing.
![]()
Figure 5. Shows the sequence graphically. Conclusion
The two studies reported here provide a detailed examination of the strategies used by untutored students working in single-sex dyads to produce a design proposal. Analysis of the data shows that significant differences exist between the strategies used by novice designers and the theoretical models contained in many textbooks and curriculum documents.
The results suggest that teachers must think carefully about the way in which students are expected to explore, develop, and communicate their design proposals, and that teaching any form of linear design process may be counter-productive to students' success in developing a solution to a design-andmake task. The studies also high light the need to identify ways in which students can be taught effectively to use metacognitive skills and thus enhance their capability as designers and makers. Teachers also need to consider how the tacit strategies students bring to the design and technology classroom may be used as a foundation for the development of capability. It would be a mistake to discount, ignore, or de-value students' existing knowledge, derived from their everyday experience, of how to design and make.
This article is based on presentations made at the International Conference on Design and Technology Educational Research and Curriculum Development (IDATER98) at Loughborough University and The International Working Seminar for Scholars in Technology Education at George Washington University. Suggestions and comments based on this paper are welcomed and should be sent to the first author at the following address: Queen's University, Faculty of Education, Kingston, Ontario, Canada, K7L 3N6. Tel: 613-533-6000 x77867. Email: Welchm@educ.queensu.ca
Dr. Malcolm Welch is an assistant professor in the College of Technological Education at Queens University in Kingston, Canada, and a Member-At-Large of Epsilon Pi Tau.
Hee Sook Lim was a Master of Education candidate and a research assistant at the Faculty of Education in the College of Technological Education at Queen's University in Kingston, Canada.
References
Akin , O. (1978). How do architects design? In J-C Latombe (Ed.), Artificial intelligence and pattern recognition in computer-aided design (pp. 65-104). New York: North-Holland.
Bennett , N. (1992). Managing learning in the primary classroom . Stoke-on-Trent, UK: Trentham Books.
Breckon , A. (1995). Data annual conference address. Design & Technology Teaching , 28 (1), 11-16.
Bruner , J. S. (1966). Toward a theory of instruction . Cambridge, MA: Harvard University.
Bruner , J. S. (1986). Actual minds, possible worlds . Cambridge, MA: Harvard University.
Chi , M. T. H., Glaser, R., & Farr, M. J. (1988). The nature of expertise . Hillsdale, NJ: Erlbaum.
Darke , J. (1979). The primary generator and the design process. Design Studies , 1 (1), 36-44.
De Miranda, M. (1998). Technology education and the cognitive sciences: A powerful connection between theory and practice . Paper presented at the International Working Seminar for Scholars in Technology Education, Washington, DC.
Eastman , C. M. (1970). On the analysis of intuitive design processes. In G. T. Moore (Ed.), Emerging methods in environmental design and planning . Proceedings of the Design Methods Group First International Conference (pp. 21-37). Cambridge, MA: MIT Press.
Elmer , R. (1996). Whose need? A study of the reflective practice of eight students on a BA honours design and technology course . Unpublished doctoral dissertation, University of Reading, UK.
Ericsson , K. A., & Simon, H. A. (1984). Protocol analysis: Verbal reports as data . Cambridge, MA: The MIT Press.
Harding , R. (1995). Professional designers in primary schools: How successful are they at teaching children design skills? Design and Technology Teaching , 28 (1), 19-21.
Hayes , J. R., & Flower, L. S. (1980). Identifying the organization of writing processes. In L. W. Gregg & E. R. Steinberg (Eds.), Cognitive processes in writing (pp. 3-30). Hillsdale, NJ: Erlbaum.
Hennessy , S., McCormick, R., & Murphy, P. (1993). The myth of general problem-solving capability: Design and technology as an example. The Curriculum Journal , 4 (1), 73-89.
Johnsey , R. (1995a). The design process: Does it exist? A critical review of published models for the design process in England and Wales. International Journal of Technology and Design Education , 5, 199-217.
Johnsey , R. (1995b). The place of the process skill making in design and technology: Lessons from research into the way primary children design and make. In J. S. Smith (Ed.), IDATER95: International Conference on Design and Technology Educational Research and Curriculum Development (pp. 15-20). Loughborough, UK:Loughborough University of Technology.
Jones , J. C. (1970). The state of the art in design methods. In G. T. Moore (Ed.). Emerging methods in environmental design and planning . Proceedings of the Design Methods Group First International Conference (pp. 3-9). Cambridge, MA: MIT Press.
Kimbell , R. (1990). Design and technology starters: Teaching and learning procedures, issues for INSET (video recording). Kent, UK: Kent County Council.
Kimbell , R. (1997). Assessing technology: International trends in curriculum and assessment . Buckingham, UK: Open University.
Kimbell , R., Stables, K., Wheeler, T., Wosniak, A., & Kelly, V. (1991). The assessment of performance in design and technology: Final report . London: Schools Examination and Assessment Council.
Lawson , B. (1990). How designers think: The design process demystified (2nd ed.). London: The Architectural Press.
Levi -Strauss, C. (1968). The savage mind . Chicago: University of Chicago.
Meyer , K. (1991). Children as experimenters: Elementary students' actions in an experimental context with magnets . Unpublished doctoral dissertation, University of British Columbia, Vancouver, Canada.
Outterside , Y. (1993). The emergence of design ability: The early years. In J. S. Smith (Ed.), IDATER93: International Conference on Design and Technology Educational Research and Curriculum Development (pp. 43-49). Loughborough, UK: Loughborough University of Technology.
Presseisen , B. Z. (1987). Thinking skills throughout the curriculum: A conceptual design . Bloomington, IN: Pi Lambda Theta.
Resnick , L. B. (1987). Education and learning to think . Washington, DC: National Academy.
Roden , C. (1995). Young children's learning strategies in design and technology. In J. S. Smith (Ed.), IDATER95: International Conference on Design and Technology Educational Research and Curriculum Development (pp. 20-27). Loughborough, UK: Loughborough University of Technology.
Schn , D. A. (1983). The reflective practitioner: How professionals think and act . New York: Basic Books.
Schn , D. A. (1987). Educating the reflective practitioner: Toward a new design for teaching and learning in the professions . San Francisco: Jossey-Bass.
Schoenfeld , A. H. (1987). What's all the fuss about metacognition? In A. H. Schoenfeld (Ed.), Cognitive science and mathematics education (pp. 189-215). Hillsdale, NJ: Erlbaum.
Simon , H. A. (1973). The structure of ill-structured problems. Artificial Intelligence , 4, 181-201.
Siraj -Blatchford, J. (1993). Constructing design and technology: An early years perspective. International Journal of Technology and Design Education , 3 (3), 19-30.
Stables , K. (1997). Out of the mouths of babes: Creative thinking and children designing . Paper presented at the Third International Conference on Creative Thinking, Malta.
Sternberg , R. J. (1994). Thinking and problem solving . San Diego: Academic Press.
Turkle , S., & Papert, S. (1990). Epistemological pluralism: Styles and voices within the computer culture. In I. Harel (Ed.), Constructionist learning: A 5th anniversary collection of papers reflecting research reports, projects in progress, and essays by the Epistemology and Learning Group (pp. 345-377). Cambridge, MA: MIT Media Laboratory.
Vygotsky , L. (1986). Thought and language . Cambridge, MA: MIT Press.
Welch , M. (1996). The strategies used by ten grade 7 students, working in single-sex dyads, to solve a technological problem . Unpublished doctoral dissertation, McGill University, Montreal, Canada.
Welch , M. (1998). Students' use of three-dimensional modelling while designing and making a solution to a technological problem. International Journal of Technology and Design Education , 8 (3), 241-260.
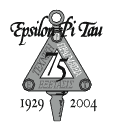

TS